X-RAY MICROSCOPY OF BIOLOGICAL SAMPLES
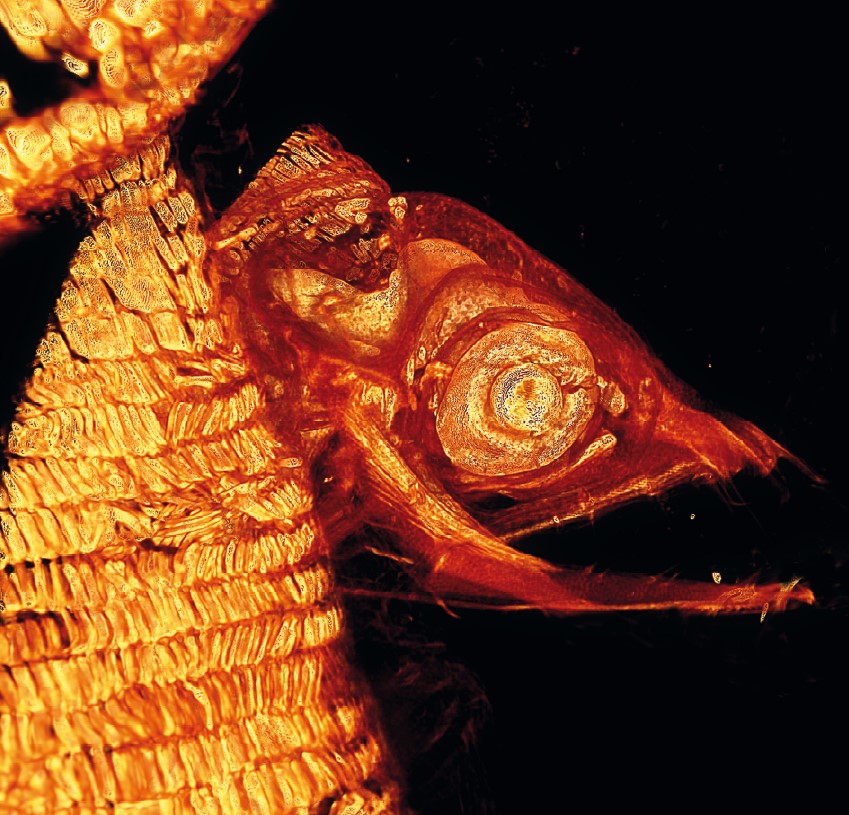
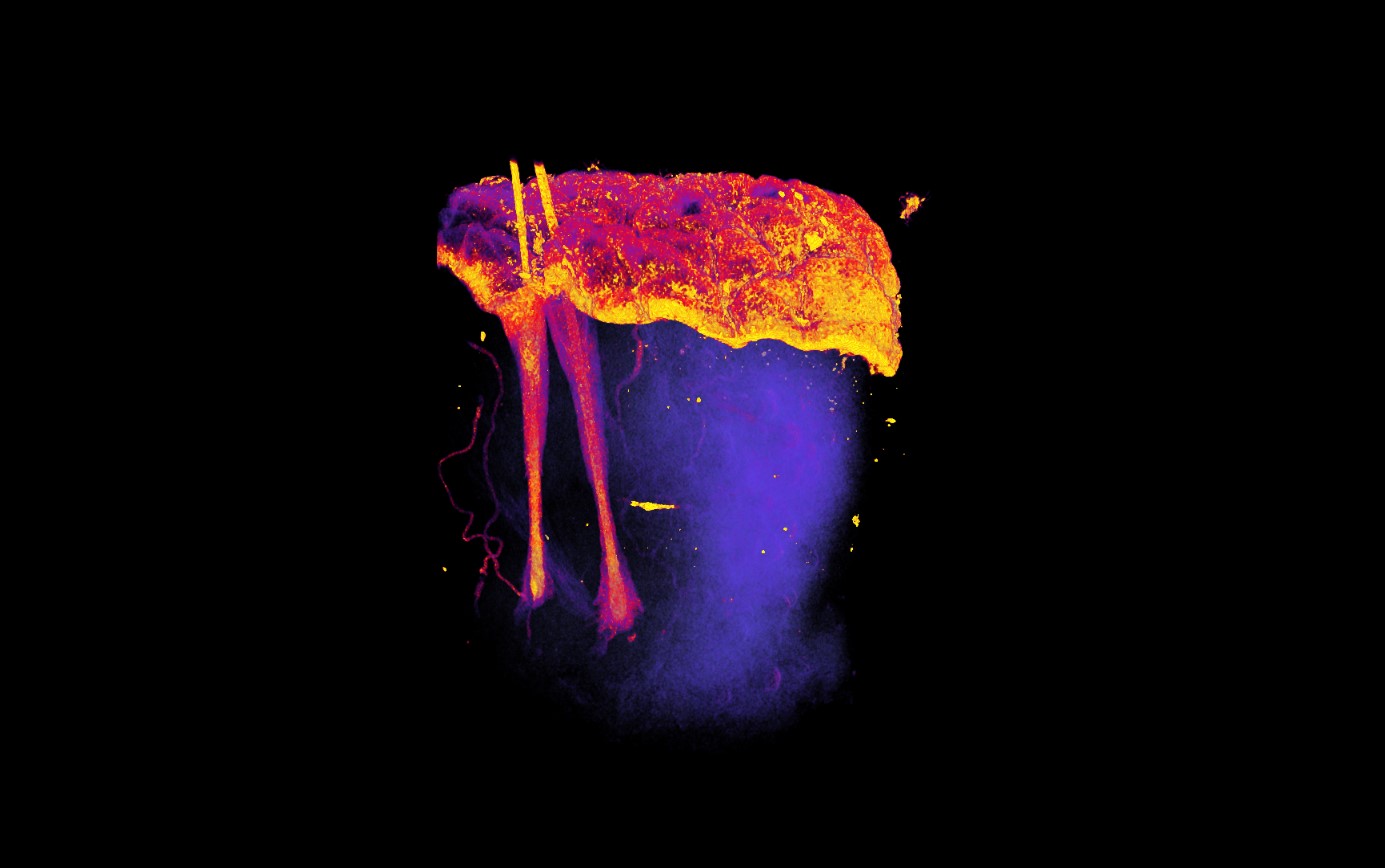
The three-dimensional cultivation of cells has revolutionized our understanding of cellular behavior both in cell culture and in vivo. To date, however, there is no imaging method that provides high-resolution volumetric data with cellular resolution in a simple and non-destructive manner. The method of reconstructing a volume from several histological sections is common, but also complex and contains errors.
X-ray microscopy, which is currently being established by the Cell Technology working group at the Fraunhofer EMB, offers a solution. This method, which is routine in semiconductor technology, has received little attention in the life sciences. X-ray microscopy can be used to achieve cellular resolutions and to clarify the three-dimensional arrangement in tissues and cell aggregates. Basically, an X-ray microscope works like a slide projector. The object is illuminated and its amplitude image is thrown onto a screen or detector (Figure 1).
The X-ray microscope consists of an X-ray source, a sample holder, imaging optics, an image detector and an optical bench for aligning the components. The intensity of the X-ray source determines the exposure time required to take an image with a good signal-to-noise ratio. The sample is fixed in the sample holder, which can be moved in a motorized manner in order to be able to position the sample precisely in the X-ray beam.
This allows the desired image area and the magnification to be selected. The X-ray detector is used to convert the X-ray intensity distribution in the image plane into a visible image.
The heart of the detector is a scintillator crystal that converts the X-rays into visible light, which is then magnified by a lens and registered by a CCD camera. In order to obtain a three-dimensional image of the sample, it is rotated in the beam path.
During this tomography, several hundred to a thousand projections are created, which are reconstructed into a three-dimensional (xyz) data set by back-projection into the original sample volume. The performance of this method for biological samples is to be demonstrated here using the larva of an eel (0.5 cm) as an example (Figure 2).
X-rays penetrate deeper into matter than visible light. This allows them to image the inside of the sample, which is opaque to visible light. In addition, much higher optical resolutions can be achieved with an X-ray microscope than with a light microscope.
The wavelength of X-rays is considerably shorter than that of visible light. Thus, the physical limit of the achievable resolution due to diffraction lies at significantly higher resolutions than with conventional light microscopes. The contrast mechanism with which X-ray images are created is a simple amplitude contrast. X-rays are absorbed differently by different materials. Where many X-rays “get stuck”, the image appears dark, while other areas are penetrated unhindered and remain bright.
If you use a staining method with X-ray absorptive elements (usually heavy metals), organic material can also create a contrast in the X-ray microscope. In this case, the previously fixed eel larva was stained with phosphotungstic acid. This staining method is not only simple and non-toxic, but also provides excellent contrast and precise differentiation of different tissues.
In this study, the elucidation of the anatomy at this larval stage was of interest. In particular, the head with the fangs, which are still present at this stage, could be clearly visualized with a 4x lens and a voxel resolution of 1.6 μm. The other native structure of the larva could also be easily worked out, such as the vitreous bodies of the eyes, the different chambers of the brain, the throat, the heart and the entire musculature.
The example of X-ray microscopy of human skin further illustrates the potential of this method for the life science area. The sample is a biopsy of the abdominal skin, which was first fixed in 4% paraformaldehyde and then stained with iodine. Sample preparation is just as quick, straightforward and non-toxic as that of the larva of the eel. The iodine diffuses quickly into the tissue and provides good contrast in the X-ray beam, so that all structures in the skin are clearly visible (Figure 3).
The epidermis can be clearly distinguished from the dermis, in which hair follicles, sweat glands, muscles and fat tissue can be seen. The recording took two hours at a resolution of 2.8 μm and therefore not only provides much faster, but also significantly more accurate data compared to other methods.
The Cell Technology group is currently establishing a method for staining individual cells and making them visible using X-ray microscopy. Recording at the cellular level offers the possibility of supporting the 3D cultivation of cells in matrices or tissue engineering.